At the intersection between nanophononics and topology, groundbreaking technological advancements are on the horizon. Physicists develop strategies to confine ultra-high frequency acoustic phonons and higher harmonics supporting topological properties.
Understanding and manipulating the topological properties are key to designing new materials with specific characteristics tailored for various applications, including electronics, energy storage and data processing. In material science, topology predicts unique properties at the interface between two materials with different topological attributes, such as robustness against imperfections. In the context of nanophononics, this ever-evolving field explores hypersound—acoustic waves spanning from gigahertz to terahertz frequencies—at the nanoscale, and offers a promising avenue for technological advancements. When delving into the complexities of nanophononics, leveraging the potential of topology in ultra-high frequency sound waves remains largely unexplored.
A group of researchers from the Centre de Nanosciences et de Nanotechnologies – C2N (CNRS, Universite Paris-Saclay, France), led by Dr. Daniel Lanzillotti-Kimura, have explored topological properties of ultra-high frequency acoustic phonons and higher harmonics in a theoretical paper recently published in Physical Review B. “We are excited to introduce topological interface states of sound waves at ultra-high frequencies, and their higher harmonics”, said Dr. Cardozo de Oliveira, co-author of the work. “This is a pivotal breakthrough for the development of lossless data processing and efficient quantum computing technologies,” he completed.
Acoustic mirrors, the simplest devices in this study, are formed by periodically stacking multilayers of two contrasting acoustic impedance materials. Within specific frequency ranges, these materials create bandgaps where waves cannot propagate, resulting in highly reflective regions. At the higher harmonics of the fundamental frequency, the structures can also exhibit high reflectivity, determined by the thickness ratio of the two composing materials. The researchers propose manipulating the thickness ratio of the periodic arrays to generate topological interface states at these higher harmonics.
An important concept in 1-dimensional topological structures is the band-inversion. The acoustic states at the edges of the bandgaps have opposite mode symmetries. By gradually changing the thickness ratio of the two materials it is possible to control the opening and closing of the higher order bandgaps. The number of openings is determined by the bandgap order. After each bandgap reopening, the edge modes undergo symmetry inversion. By concatenating two acoustic mirrors with different mode symmetries, the topological interface state is generated.
By stacking two distinct acoustic mirrors with overlapping bandgaps, under specific configurations, the acoustic vibrations become confined at the interface of these mirrors, leading to the generation of topological interface states. These states, arising from the unique properties at the boundary of materials with disparate characteristics, display remarkable robustness against external disturbances. “Through properly engineering the higher order bandgaps, we have not only demonstrated the support for interface states across different harmonics, but have also paved the way for the realization of multi-channel protected states”, said Dr. Cardozo de Oliveira. This groundbreaking achievement marks a significant stride in the intricate landscape of data processing, promising a harmonious future where information can be processed and communicated with unprecedented efficiency and reliability.
Source: “Topological nanophononic interface states using high-order bandgaps in the one-dimensional Su-Schrieffer-Heeger model,” by Anne Rodriguez, Konstantinos Papatryfonos, Edson Rafael Cardozo de Oliveira and Norberto Daniel Lanzillotti-Kimura, Physical Review B, 108, 205301 (2023).
The article can be accessed here.
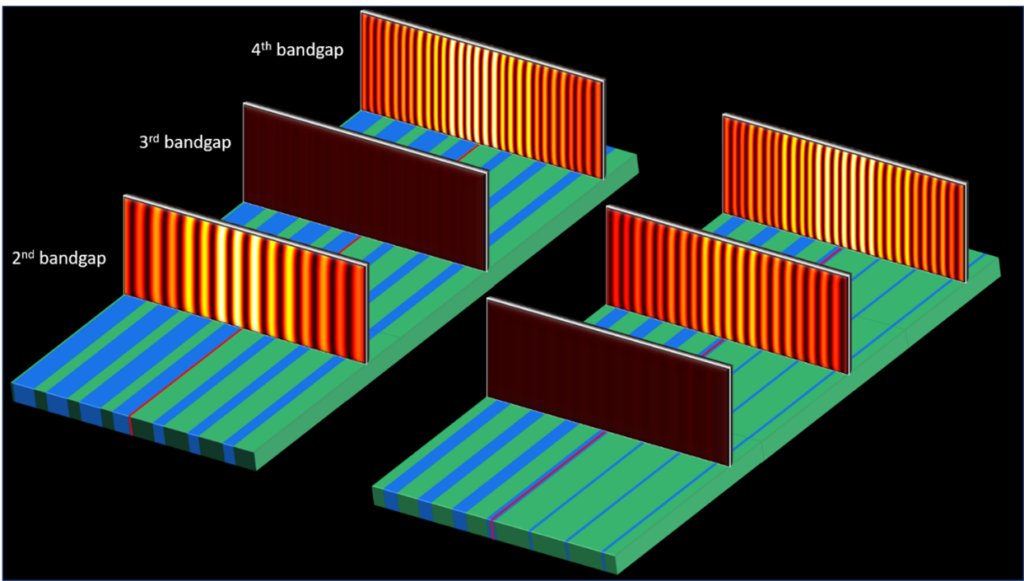